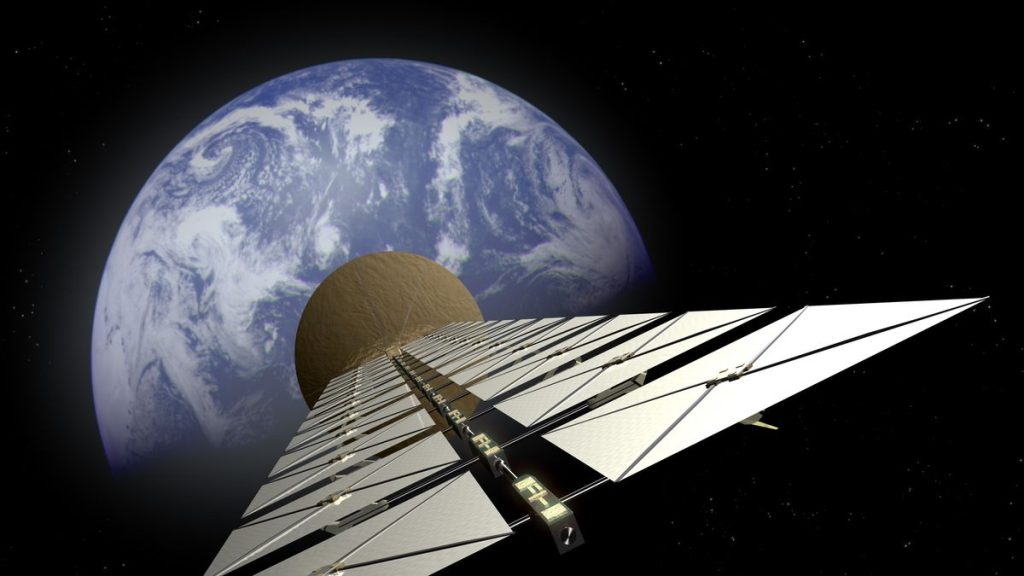
4 futuristic space technologies — and when they might happen (Image Credit: Space.com)
World Space Week this year, which runs between Oct. 4 and Oct. 10,is celebrating how space technology is aiding us in our fight against climate change on Earth — but sometimes it pays to also look outward at what technology can offer us as we expand into space to harness the energy and the worlds that lie out there.
Here we highlight four technologies, look at the challenges that they pose and give some indication of when they might come to fruition — if ever. The time estimates are not necessarily a prediction of when they might happen, but are intended to give a rough idea of how much work still needs to be done on them.
Related: Space-based solar power may be one step closer to reality, thanks to this key test
2040s-2050s: Space solar farms
Currently, solar power provides just over 5% of the world’s total electricity supply, but we can do much better than that.
The best place to feel the sun’s energy is in space, without clouds to block the view or an atmosphere to absorb our star’s rays. A huge array of solar panels would therefore have an unfettered view of the sun, but the tricky part of this idea concerns building such a space-based array in the first place. Plus, even if we manage that somehow, how would we get the harvested solar energy down to Earth?
Compared to most technologies on this list, power beaming from space is actually ahead of the curve. In January of 2023, the Caltech-built Space Solar Power Demonstrator launched into Earth orbit. On board was an instrument called MAPLE, the Microwave Array for Power-transfer Low-orbit Experiment. MAPLE successfully converted solar energy into microwaves and then beamed the microwaves down to a receiving station at Caltech, where it was converted into electricity. It was a pretty low amount of power — just milliwatts — but it was an exciting proof of concept.
Now, the Japanese Aerospace Agency, JAXA, working with commercial interests, are exploring their own program that the agency hopes to culminate in a solar farm capable of producing one gigawatt of energy and beaming it down to Earth. However, building a solar farm isn’t easy.
A solar panel in space, above the absorbing effects of the atmosphere, receives about a kilowatt of energy per square meter (10 square feet) of the sun. Solar panels are not 100% efficient, however; currently available commercial models have an efficiency of just 30%, meaning a solar panel in space can realistically produce just 300 watts per square meter. To scale this up to producing 1 gigawatt of energy, which would be the equivalent of a nuclear power station on Earth, would require an enormous array of solar panels, multiple kilometers in diameter, with a mass of 10,000 metric tons. Compare this to the International Space Station, which has a mass of 419 metric tons, and that shows just what a daunting engineering task this would be.
Supposing a solar farm could be built in space, it would be placed in geosynchronous orbit, 35,786 km (22,236 miles) above the Earth. The challenge would then be to keep the microwave beam narrow and on target — you wouldn’t want the microwave beam to stray and fry something accidentally. Although lasers instead of microwaves would be easier to direct, laser energy can be absorbed by water vapor in the atmosphere or blocked by clouds, whereas microwaves pass freely through them.
Lasers, though, might be more suitable for space-to-space power transfer. This could extend the life of satellites, for example, but they would have to be built with some kind of receiver to accept the incoming laser power beam. We could also imagine a network of solar farms and relay satellites around the moon, beaming power via lasers to a lunar base on the surface.
Second half of the 21st century: Space elevators
This is an old science fiction concept, first conceived by Russian scientist Konstantin Tsiolkovsky — rather than blasting off in a rocket atop a dangerous column of flame, why not ride into space on an elevator car?
The basic design of a space elevator sounds simple. A thick cable extends from a location within 10 degrees of the Earth’s equator up into space. The forces acting on the cable would be fierce, with Earth’s gravity trying to pull it down, and the centrifugal force on a mass at the end of the cable in Earth orbit pulling it the other way, keeping it taut. The stresses and tension of this cable would be so great that it would need to be made from a material 50 times stronger than steel, however. The only material strong enough are carbon nanotubes, which are “grown” from a process of chemical vapor deposition. The problem is, as the name suggests, carbon nanotubes are small, and the longest grown have been about 14 centimeters (5.5 inches) — that’s a big difference to the 100,000-kilometer (62,000-mile) length of a space elevator
Suppose, though, a way is found to manufacture longer nanotubes and mass produce them. Once we have the materials, Stephen Cohen of Vanier College in Quebec, Canada, who is author of “Getting Physics: Nature’s Laws as a Guide to Life” and an expert in space elevator design, thinks that the subsequent design phase would take five to 10 years, in parallel with the maturing of the cable material.
“The cable would be spooled down from geo orbit and eventually fastened to Earth port,” Cohen told Space.com. “The ship that unspools it would use fuel to gain altitude and become the far-end anchor some 100,000 kilometers away. Alternatively, a separate ship transports the cable portion above geosynchronous orbit while the original vessel assumes the role of a geo port, which is the true ground central for long term operations. This deployment process could easily take a year.”
While we’re waiting on the carbon nanotubes, Zephyr Penotre of the University of Cambridge in the U.K. and Emily Sandford of Columbia University in New York think we could be setting about building a space elevator from the moon with current technology. Because of the moon’s slower spin rate and lower gravity, they reason that the tension felt by a lunar elevator would not be as great as an elevator coming up from Earth and that carbon polymers such as Zylon would do the trick.
Penoyre and Sandford call their concept a “space-line.” Whereas an elevator from Earth would be quite chunky, perhaps ferrying multiple elevator cars up and down simultaneously, the space-line would be a thin wire with a total mass of 40 metric tons. Anchored on the lunar surface, it would dangle toward Earth, stopping somewhere around geosynchronous orbit. So, it wouldn’t actually touch Earth or subject it to the destructive tension that doing so would incur.
The idea is that a mission to the moon would launch to geosynchronous orbit, rendezvous with the space-line and ride it the rest of the way to the moon, or to a base at the Earthmoon Lagrange point where the gravity of the Earth and the moon balance out. It would cut the amount of fuel needed to get to the moon by a third; a mission would still have to launch out of Earth gravity well, but the space-line would be solar powered.
Penoyre and Sandford estimate that a prototype would cost billions of dollars, but in the long run it would save money if we are to permanently settle on the moon.
Cohen is looking even further afield, to Mars. “I think a first Mars venture with humans precedes space elevator construction but I would argue that no sustainable colonization of Mars is achievable without an infrastructure like the space elevator,” he said. “And so, design and construction phases of the space elevator should probably coincide with the first humans on Mars.”
Currently, there are no concrete plans for humans to travel to Mars. Much might depend upon how NASA’s Artemis lunar program develops. If a lunar base can be built and crewed by the 2030s, astronauts can learn the skills they’ll need to survive on the Red Planet, but first we need to learn how to live on the moon. And to do that, we’ll need power, as we’ll next discover.
2030s: Nuclear power on the Moon
The moon is tidally locked to the Earth, which means that we always see its same face, the familiar “Man in the Moon.” Don’t be fooled into thinking that the moon doesn’t rotate, however — it does, at the same rate that it revolves around our Earth, which is how it always keeps the same face directed towards us. It takes a little over 27 days — about four weeks — to rotate once, which means (almost) everywhere on the moon sees two weeks of daylight and two weeks of night. The exception is some areas very near the poles, which can see 80% daylight. However, if a lunar base were to be solar powered, it would need 100% daylight, so an alternative power source is needed.
Nations and space agencies around the world are now racing to develop the first nuclear fission reactors for the moon. In February, NASA and the U.S. Department of Energy selected three design proposals for a fission reactor to fly on a future Artemis mission. The reactor has the design specification of producing 40 kilowatts for at least 10 years on the lunar surface.
Meanwhile, the Russians and the Chinese have announced that they are jointly planning an International Lunar Research Station with a nuclear reactor, to launch between 2033 and 2035. However, they admit they have not yet come up with a way of cooling the reactor.
“The major issue is what to do with all that waste heat since there is no air on the Moon to convect it away,” Simon Middleburgh of the Nuclear Futures Institute at Bangor University in Wales, told Space.com.
Middleburgh is working with the U.K. Space Agency and Rolls-Royce to develop a nuclear fission reactor that could fly to the moon on a future mission. Rolls-Royce have considerable experience working with nuclear reactors, since they outfit the U.K.’s nuclear submarines with them.
“The aim for the reactor energy output would be of the order of 100–300 kilowatts in combined heat and electrical power – both of which would be extremely useful up there [on the moon],” Middleburgh said. “This is an enormous amount of power compared to previous missions, and as the site [for a lunar base] grows, we may want to build a second or third system that will also provide assurance of energy supply. But we won’t be building 100 megawatt systems any time soon.”
2070s-2120s: Interstellar travel
It took nine years for the fastest ever space mission, NASA’s New Horizons, to reach Pluto at a distance of 34 astronomical units (AU) from the sun. For context, an astronomical unit is the average distance between Earth and the sun. The closest star to the sun is Proxima Centauri. It’s 268,779 AU (4.2 light-years) from the Sun. Having passed Pluto, New Horizons reached a velocity of 84,000 km per hour (52,000 mph) and it would take about 80,000 years to reach the distance of Proxima Centauri.
Clearly, we’re going to need to find a faster way if we ever hope to travel between the stars, but there are people working on the problem. In the 1970s, members of the British Interplanetary Society developed Project Daedalus, which was plans for a two-stage nuclear-fusion powered but uncrewed starship that could ultimately reach 12% of the speed of light.
In 2016, the Breakthrough Foundation initiated Project Starshot, the concept of which is to drive a fleet of tiny spacecraft, called StarChips, attached to light sails that are pushed up to 20% of the speed of light by powerful laser. They would reach Proxima within decades rather than centuries. Just one problem though: the laser energy output must be up to 100 gigawatts — the equivalent of 100 nuclear power stations.
To solve this problem, we could combine futuristic technologies — the solar farms that could be built in orbit to harness solar energy could instead be used to power the lasers. It’s all technology we have now, but on a scale far in advance of what we currently have. The stars are there waiting for us — but it seems they might have to wait a little while longer.
Right now, all we have is Earth.
This article is part of a special series by Space.com in honor of World Space Week 2024, which runs from Oct. 4 to Oct. 10. Check back each day for a new feature about how space technology intersects with climate change.