New research has shed doubt upon the nature of a distant “dead star” that exists in a binary system with an active stellar companion. Scientists behind the research think this stellar corpse is actually a monster-sized white dwarf.
The discovery raises the question of how the binary’s “living” star has managed to survive in close proximity to such a stellar corpse without being dragged towards it and violently consumed. The study is detailed on the pre-print website Arxiv.org and has been submitted to the Open Journal of Astrophysics.
In 2023, astronomers discovered this star located 417 light-years from Earth has a shadowy companion, believed to be the “dead star.” Initially, scientists thought the stellar remnant in the binary system, LAMOST J2354, which received its name because it was discovered by astronomers using the Large Sky Area Multi-Object Fiber Spectroscopic Telescope (LAMOST), was a neutron star. However, this team has discovered some clues that throw that identification into doubt, suggesting this lurking dead star is a larger-than-average white dwarf.
“The J2354 system was discovered by the LAMOST team and is claimed to host a neutron star,” team leader Michael A. Tucker, a researcher at the Center for Cosmology and Astroparticle Physics, Ohio State University (OSU), told Space.com. “I was originally interested in this system because if it was a neutron star, it likely formed during a core-collapse supernova.
“The nearby explosion of the massive star, the neutron star progenitor, would have impacted the companion and dumped lots of metals onto the surface,” he added.
The system is nearby and bright, relatively speaking, meaning it provides an excellent opportunity to actually look for this expected “pollution” from the supernova, Tucker explained.
“Unfortunately, we didn’t find any, which is one of the reasons we ended up favoring a white dwarf over a neutron star,” he said.
Related: Scientists find slowest spinning ‘radio neutron star’ — it breaks all the dead-star rules
White dwarf or neutron star: What’s the difference?
White dwarfs and neutron stars have many similarities, but there are also many differences between these two classes of stellar remnants.
The main similarity is that both types of stellar remnants are born when stars exhaust the fuel for nuclear fusion at their cores and can no longer support themselves against the inward push of their own gravity. This results in the star collapsing and creating ultradense matter and a remnant that is protected against further collapse by an aspect of quantum physics that prevents particles of the same type from cramming too close together.
White dwarfs are born when stars with masses around that of the sun exhaust the hydrogen in their cores. In around 5 billion years, the sun will undergo this process, leaving a smoldering cosmic ember white dwarf at the heart of what remains of the solar system.
However, when this depletion of hydrogen happens for a star around eight times more massive than the sun, the collapse of the star creates the pressure and temperature to begin the nuclear fusion of helium in the core to heavier elements. This continues until the star has a core of iron, an element that no star can fuse into heavier elements.
The collapse of the star at this time triggers a supernova explosion as long as the star still has enough mass to exceed the so-called Chandrasekhar limit, which is around 1.4 times the mass of the sun. The result of this fusional collapse is the creation of a neutron star, a stellar remnant that crams between one and two times the mass of the sun into the width of the average city on Earth.
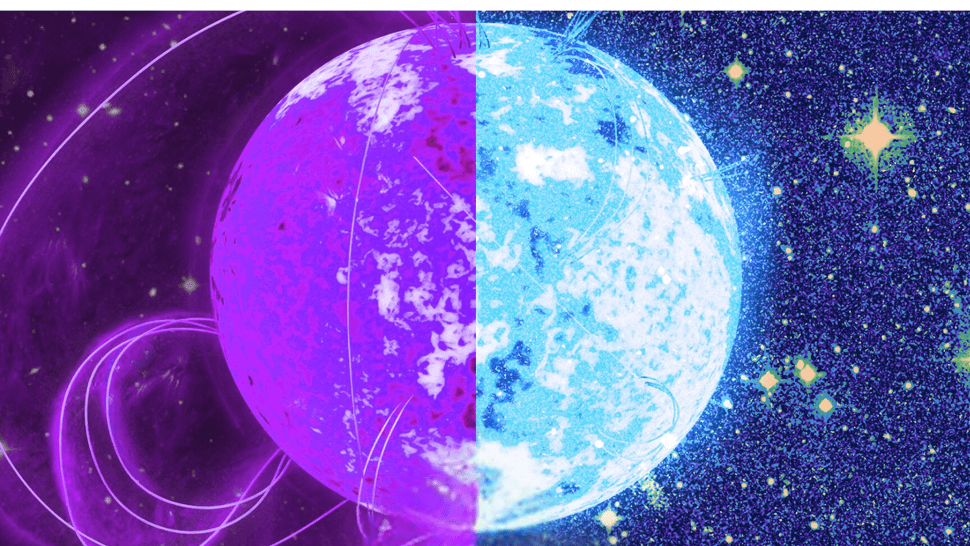
The dead stellar companion in the LAMOST J2354 binary system was initially identified as a neutron star because its mass seems to be right around the Chandrasekhar limit.
“The tricky bit is that the mass of ‘heavy’ white dwarfs overlaps with the mass of ‘light’ neutron stars,” Tucker said. “So, even knowing the mass exactly does not answer the question of if this hidden companion is a white dwarf or a neutron star.”
The team was able to find some clues that indicate that the dead star of LAMOST J2354 isn’t a neutron star. The first of these clues was the fact that the binary system’s living star doesn’t contain the wreckage or “pollution” that would normally showered upon it by the supernova cosmic explosion that accompanies the death of a massive star and the birth of a neutron star.
This pollution could be absent if the companion star was further away when the supernova occurred, but Tucker explains that were this the case, then the neutron star would have had to have been “kicked” inward toward its companion to create the orbit observed today.
“Orbits typically expand after a supernova because you have lost mass from the system, so producing a closer orbit requires a finely-tuned kick direction and velocity – we estimated a chance probability of only a few percent that this would happen,” Tucker said.
Additionally, if this were a binary of an ordinary star orbited by a neutron star, it would be twice as close to Earth as the next nearest system of the same nature. That would indicate the density of such systems in the Milky Way is around eight times higher than currently predicted. That means current models favor a 1.4 solar mass white dwarf in LAMOST J2354.
“It is not the most massive white dwarf known, but it is significantly above average,” Tucker continued. “The more massive the white dwarf, the more difficult it is to see/find, generally speaking.”
He added that this is because white dwarfs have the unique trait of becoming smaller in size as the mass increases, so massive white dwarfs are smaller and fainter than less massive white dwarfs.
“This is compounded by the fact that more massive white dwarfs also cool faster than lower-mass white dwarfs due to the higher densities increasing the conduction efficiency, meaning it’s easier for energy to move from the core to the surface and escape,” Tucker said. “As a result, massive white dwarfs are smaller, colder, and therefore much fainter than lower-mass white dwarfs of the same age.”
How did star escape its lurking zombie companion?
For Tucker, the most interesting aspect of LAMOST J2354 is its evolutionary history.
“The current orbit is very, very close [around 1.5 times the width of the sun], so the system certainly experienced one common-envelope event,” he explained.
The “common envelope” stage is a common feature of the evolution of binary stars because when the more massive star runs out of hydrogen in its core, it evolves into a red giant. If the binary is separated by less than 500 times the width of the sun, the companion star of the red giant becomes embedded within its extended, tenuous layers, hence the phrase “common envelope.”
“There is drag and friction at work, so the orbit slowly shrinks as the envelope is ejected from the system. Despite this process affecting nearly every aspect of binary star evolution, it is extremely complicated to model due to the ridiculous time and spatial scales involved,” Tucker continued. “The result of a common-envelope phase is closer binary, and the more massive primary star has been stripped of most of its envelope, leaving behind a ‘helium star‘ or a ‘sub-dwarf’ star.”
For low-mass primary stars, less than three times as massive as the sun, Tucker said that helium is slowly fused to carbon and oxygen in the core before the binary evolves directly to a normal star and a “regular” white dwarf binary.
“However, more massive primaries with over three times the mass of the sun can ignite helium burning in a shell around the carbon/oxygen core,” he explained. “This produces a second giant phase where the stars puff back up to a few hundred times the width of the sun and start a second common-envelope phase. Again, this should reduce the orbit at the expense of ejecting the primary star envelope.”
What the team really wants to know is how the low-mass companion in such a binary experienced two separate common-envelope phases without spiraling into a merger with the primary dead white dwarf star.
“We know binaries merge as most massive white dwarfs show evidence for mergers due to their very rapid rotation, so how/why did this one survive?” Tucker asked. “I pitched this research to the binary-star theorists as a way to test our ideas of how common-envelope evolution governs the outcome of close binaries.”
At the moment, though the team’s findings suggest a massive white dwarf lurking in this system, the possibility of a neutron star still can’t be ruled out.
“The best way to actually confirm the companion is a white dwarf with an ultraviolet spectrum. Unfortunately, that can only come from the Hubble Space Telescope, which at this point is difficult to obtain observing time with,” Tucker concluded. “Our proposal for this target was actually rejected last year, so we went ahead and published what we had. We will try again in future cycles but Hubble has begun to show its age and to degrade.”
That means it may be some time before astronomers know for sure if the dead star in LAMOST J2354 is a neutron star or a massive white dwarf.
The team’s research is published on the paper repository arXiv.